What are the types and compositions of dye? Information about natural and chemical dyes, their properties and uses.
DYE is matter used to impart color to other materials by dyeing. Dyes are used to color natural fibers, such as cotton, wool, silk, and linen, and synthetic fibers, such as nylon, cellulose acetate, and polyester. They are also used on other types of substances, such as leather, wood, food, paper, and in photography. Dyes for home use are the same as those used in industry, and they are applied by the same methods.
Dyes and Other Colored Substances.
Not ,all colored compounds will behave as dyes; for example, indigo and cochineal are dyes, but red iron oxide and ultramarine are not. In the dyeing process a solution of the dye is made, and the material to be dyed, called the substrate, is put in the solution. Colored matter from the solution is transferred to the substrate by the process of absorption; the removal of the dye from the solution is called exhaustion. This is a general description of all dyeing processes. For a particular case dyeing assistants are added to the dye solution to facilitate the interaction of a particular type of dye with the substrate. Typical dyeing assistants include acids, alkalis, and wetting agents. Red iron oxide and ultramarine are examples of pigments. These substances are not plastics, and rubber.
The process of absorption of a dye by a substrate implies that there is a chemical or physical interaction between the material and the dye molecules, leading to the formation of bonds. The behavior of a dye is thus dependent both on its chemical structure and on the structure of the substrate.
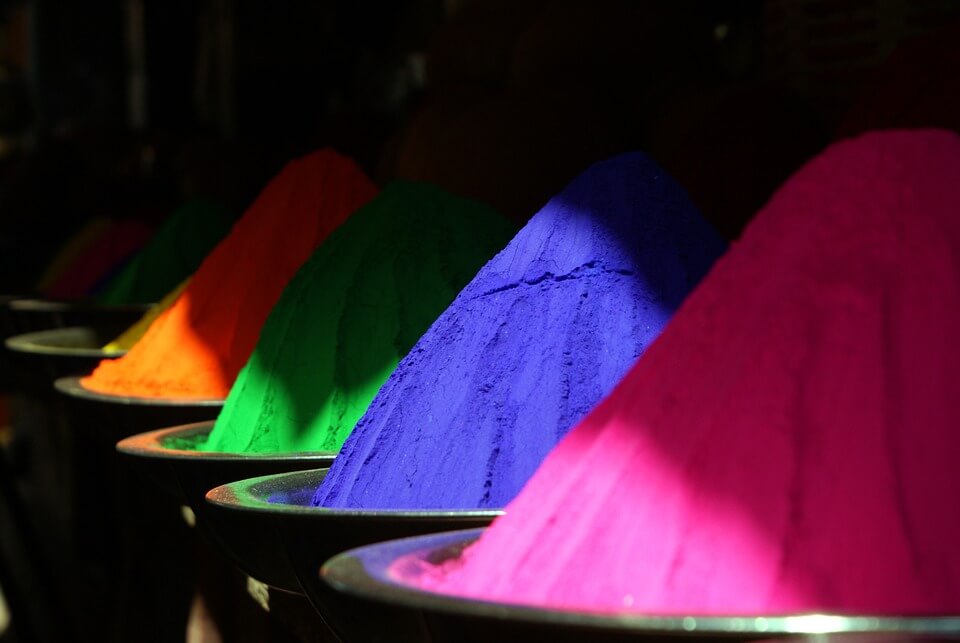
Source: pixabay.com
The Color of Dyes.
Different molecular systems absorb light at different energy levels or wavelengths. Which wavelengths are absorbed and which are reflected determine the color of an object. In terms of color in dyes, the absorption of the light energy depends on the dye’s acting as an optical filter on a molecular scale. The part of the dye molecule that is primarily responsible for this action is called the chromogen. Normally a chromogen is part of a molecule that bears substituent groups that modify the light absorption in various ways, so that a variety of shades may be obtained from a given chromogen. Thus, much of the history of dye production is concerned with the preparation of different chromogens.
The light-absorption characteristics that give organic dyes their color arise from the electron structures of the dyes. An atmosphere of delocalized electrons associated with the conjugated system of the dye molecules determines the dye color. Absorption of light energy depends upon the energy level of the electrons with which it interacts, so that different chemical structures lead to different colors.
Delocalized electrons are also involved in the development of the intermolecular forces of attraction, known as dispersion forces, that bind dye molecules to fabrics. Other kinds of intermolecular forces are also thought to be involved in binding dye molecules to fabric substrates. Such forces include coulombic or electrostatic forces between oppositely charged parts of molecules, hydrogen bonding, and hydrophobic bonding. The latter is a kind of interaction which arises from the special physical and chemical properties of water.
The study of light absorption by dyes has always aroused interest from the point of view of color prediction and calculation; light absorption by dyes has also been studied in terms of the photochemical reactions involved. The complexity of even simple dye molecules has prevented the development of a general theory relating color and molecular structure; however, a number of semiempirical relations between molecular structure and color have been developed that work well within a group of related compounds.
The calculation and prediction of colors is of great importance in industry, and a number of methods have been evolved that allow the relations inherent in color mixing to be calculated on analog or digital computers. These developments are bringing about great changes in all industries concerned with the use of color.
The Fastness of Dyes.
The problems of color fastness during washing and on exposure to light have always represented a major challenge to color chemists. The absorption of most dyes by a substrate is a reversible process. In the dyeing process, after a certain period of time an equilibrium is set up in which the rate of absorption of the dye by the fiber is equaled by the rate of desorption (loss of dye by the fiber). When the dyed fiber is washed, a new equilibrium occurs, which means that there is a net desorption of dye and a loss in depth of shade with, perhaps, the staining of other articles. This process may be limited by making dyes with large molecules that diffuse slowly from the substrate during washing; by using dyes that form strong bonds with the substrate; and by using substances, such as azoic dyes, that form insoluble pigments that cannot wash out.

Source: pixabay.com
NATURAL DYES
From prehistoric times until the mid-1800’s, when the first synthetic dye was developed, dyeing was done with natural dyes, such as indigo, saffron, and henna. A wide range of natural coloring matters were used. Some of them, such as madder root extract with an alum mordant, gave excellent results, while others were very poor.
Many of the natural coloring matters, such as logwood and madder, form slighdy soluble complexes with the ions of transition metals. This is because they contain hydroxyl and other groups that can chelate such metal ions. The early dyers found that it was possible to pretreat the textiles, or “mordant” them, with various naturally occurring salts. The mordant forms a chelated complex on the fiber in the dyeing process. Alum, an aluminum sulfate compound, is a frequently used mordant.
The use of different salts with the same plant extract dyes was found to give rise to different colors, thus providing a wider variety of shades. The shade produced by such dyes is also affected by impurities in the water supply, so that in some cases a certain shade becomes associated with particular districts or even villages.
One of the most important of the natural dyes was the coloring matter contained in the leaves of indigo-bearing plants. This substance is soluble in water but oxidizes to the insoluble pigment indigo. The pigment may then be reduced by various reducing agents, applied to the substrate from solution, and then reoxidized to produce a blue material. The preliminary reduction of the indigo is known as vatting; the dye is an early example of the vat dyes.
By the end of the 19th century a method for the commercial synthesis of indigo had been invented. Coupled with the synthesis of madder (the dye alizarin), this marked the effective end of natural dyes as commercial materials.
SYNTHETIC DYES
In 1856 at the Royal College of Chemistry in London the British chemist Sir William Henry Perkin attempted to synthesize quinine. As part of his research, Perkin oxidized aniline sulfate with potassium dichromate. Fortunately, as it turned out, Perkin’s aniline contained some toluidine as an impurity, and consequently, from the black tar that he prepared, Perkin was able to extract the first synthetio dye, mauveine.
Basic Dyes.
The first synthetic dyes fell into the class of basic dyes— that is, the colored molecules were cations (bearing a positive electrical charge). This type of dye is easily applied to materials, such as wool, silk, and nylon, that contain anionic groups (negative groups, such as carboxyl groups). Basic dyes may be employed for dyeing cellulosic materials, but because these materials contain few anionic groups, mordants must be used, which confer a negative charge to the fiber.
The commercial manufacture of mauveine in England marked the beginning of the organic chemical industry, although its technical life did not last more than a decade. It was superseded by a flood of new products from laboratories in England, France, and Germany. Other early basic dyes included magenta and rosaniline. Basic dyes are generally characterized by poor fastness to light and are relatively little used. However, modern developments have produced fast basic dyes which are of great importance in the dyeing of acrylio fibers.
Acid Dyes.
In 1862 it was observed that phenyl rosaniline could be treated with, sulfuric acid under appropriate conditions to introduce the sulfonic acid group. The dyes thus formed are anionic and are therefore termed acid dyes. The acid dyes are water-soluble and do not suffer from many of the deficiencies of basic dyes. They are used on wool, silk, and some of the synthetic fibers.
Azo Dyes.
The next major breakthrough came with the utilization of the diazo reaction in the formation of synthetic dyes in the late 1870’s. The diazo reaction had been discovered by the German chemist Peter Griess in 1858, when he observed that aromatic amines (R’NHa) could be readily converted to diazonium compounds’ (R’N = N’Cl) under appropriate conditions. Later it was found that diazonium compounds could be coupled with phenols and other aromatic molecules to form azo dyes (RN=NROH).
More than 2 million azo dyes have been prepared since their discovery. The early ones possessed excellent properties for the dyeing of wool and silk but were of litde value for cellulosic fabrics.
Some azo dyes are used with mordants. In this case, chelating metals are used with the dyes to achieve both light and wet fastness. Without the chelating group the azo group is open to photochemical attack and the chromogen can be destroyed. This possibility can be reduced considerably by incorporating a chelating system, usually the o-o’ dihydroxy azo system, into the molecule to chelate a metal atom.
The involvement of the azo nitrogen atoms in the chelate system brings about considerable stabilization against chemical and photochemical activity and gives very good light fastness. In the dyeing of cellulosic materials the use of copper as a metallizing agent for o-o’ dihydroxy azo direct dyes was developed to improve light fastness. On protein substrates such as wool, when chromium is used as the metallizing agent, high wet fastness, as well as light fastness, is also achieved because the chromium atom reacts with the wool to give an effective chemical linkage between the dye and the fiber.
The old mordanting system of pretreatment of the fiber was unsuitable for the production demands of the 20th century, and two modified techniques were developed. In the after-chrome process the dye was exhausted onto the fiber and then chelated with the metal ions in an after-treatment in the same bath; in the metachrome process the dye and potassium dichromate were applied simultaneously. The dichromate was reduced by the wool to give trivalent chromium ions, which were chelated by the dye, which was absorbed at the same time.
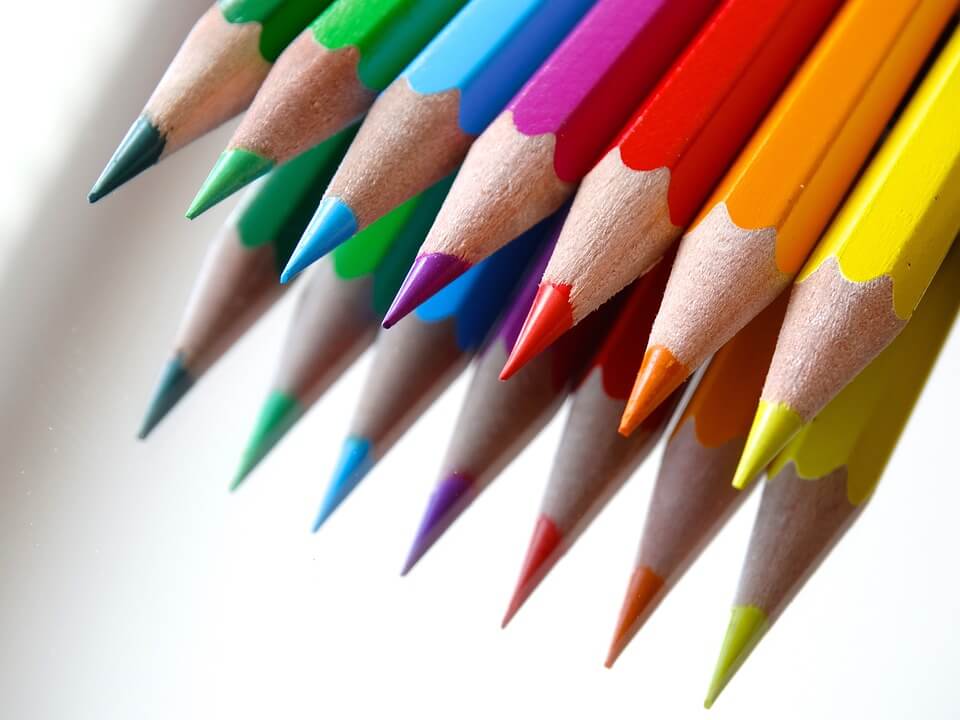
Source: pixabay.com
Direct Dyes.
By 1884, the German scientist P. BĂ´ttinger had completed investigation into the relationship between the chemical structure of a dye and its affinity, or substantivity, for cellulose; this research resulted in the introduction of the bis-azo dye, congo red. This was the first of a new class of direct dyes, which can be applied directiy to cellulose without pretreatment of the fiber with a mordant.
Azoic Dyes.
At this time attempts were made to synthesize an insoluble azo pigment directly on the fiber. An aromatic phenol was applied to cellulosic substrates, and after appropriate rinsing the fabric was treated with a diazonium salt solution to give a billiant pigmented material in which the pigment crystals were held in the fiber interstices. The first dye of this kind was vacan-ceine red, which was obtained by coupling /3-naphthol with diazotized jS-naphthylamine. There were few developments in this field until 1911, when Zitscher and Laska produced a naph-thol of significantly higher substantivity.
Vat Dyes.
From the research on indigo, derivatives of indigo were prepared, and a range of indigoid vat dyes was produced. In 1901 the German chemist René Bohn attempted to make the anthraquinone analogue of indigo by applying the indigo/sodamide fusion to the glycine derived from aminoanthraquinone. The result was a new multiple-ring quinonoid structure, indanthrone, which is a vat dye with vastly superior properties of wet and light fastness. Since that time, new synthetic vat dyes of comparable properties have been produced, so that fast dyeings on cellulosic fabrics can be produced over a wide range of shades.
Dyes for Man-made Fibers.
Many new problems in dyeing arose with the emergence of the synthetic fiber industry in the 1920’s. At that time cellulose acetate was established as a useful textile material, but its commercial future was jeopardized because it could not be satisfactorily dyed with the available dyes. The more recently fabricated fiber, polypropylene, has also presented this difficulty.
It was found that cellulose acetate could be dyed by simple aminoazo compounds with very low solubility in water, provided they could be satisfactorily dispersed in an aqueous dyebath. The production of satisfactory orange and red shades was easily achieved, but the complete solution to the problem only came about with the work of the British chemists Baddiley and Shep-erdson, who prepared suitably dispersed aminoanthraquinone blue dyes. The disperse dyes, as this new class was called, are sparingly soluble in water and actually dye from solution.
Since the 1920’s man-made fibers have been produced in increasing quantity and variety. By the mid-1960’s the consumption of man-made fibers exceeded that of wool. For the most part these fibers are particularly suitable for dyeing with disperse dyes, and considerable advances have been made in improving the fastness of such dyes.
One of the consequences of using a weakly polar dye molecule, is that the unionized dye often possesses a significant vapor pressure. An example of such a dye is C. I. Disperse Orange 15. Althought this can give rise to the problem of sublimation fastness, in which the dye may vaporize during hot pressing treatments, it has also made possible the development of dry-heat dyeing processes, which utilize the volatility of the dye. Research has explored die possibility of a completely dry-vapor phase dyeing process. Improved sublimation fastness is achieved by the use of dyes of higher molecular weight or increased polarity. These dyes are more difficult to use in dyeing because they diffuse more slowly in the fiber. However, high-temperature ( 130— 140°C) and pressure-dyeing processes and appropriate dyeing machinery have been developed to overcome this problem.
The color fastness requirements of the old cellulose acetate fibers were not stringent. This was not true of later fibers. Nylon, the next important synthetic fiber developed after cellulose acetate, can be dyed with either acid or disperse dyes. The acid dyes are used where fastness is required. However, polyester, polyacrylic, and polypropylene fibers required the development of new dyes to give fast results.
Polyester fibers cannot be dyed with ionic dyes, and since the polyester is a high-quality fiber, high fastness is required. The growing importance of this fiber has provided the main incentive for the development of sublimation-fast and wash-fast disperse dyes.
Polyacrylic fibers are anionic in character and may be dyed with both disperse ( non-ionic ) and basic (cationic) dyes. However, the fibers cannot be dyed at high temperatures, and fastness must therefore be achieved with the basic dyes. Until these synthetic fibers appeared, the basic dyes were declining in importance because of their poor light fastness. However, acrylic fibers possess the surprising property of giving dyeings of good light fastness with many basic dyes. This may be connected with the relatively high electrical conductivity of the polymer.
Polypropylene presents another problem; it is very readily dyed with disperse dyes, while ionic dyes show no significant substantivity for it. However, the disperse dyes are removed from polyDronylene almost as easily as they are applied. There has been considerable research into the production of modified polypropylenes and suitable dyes. The most successful approach so far has been to incorporate into the polymer metal ions that can chelate with suitable dyes to produce a fast dyeing.
Metal Complex Dyes.
The most significant developments in the period since 1945 have been the growing importance of 1:2 chromium complex dyes and the appearance of a completely new class of fiber reactive dyes. The 1:2 metal complex dyes, which contain two molecules of dye combined with one molecule of metal, are a logical development of the mordant dyes. It was found as early as 1925 that certain dyes that contained chelating groups formed soluble 1:1 complexes with chromium—that is, one molecule of dye combined widi one atom of metal. These dyes could be applied under strongly acid conditions to give good dyeings on wool, and they were used fairly successfully over the next two decades.
Their development stimulated further work on the use of preformed metal complex dyes for wool, and by the 1940’s investigations into the use of 1:2 complexes were fairly advanced. These dyes have a high substantivity, and in order to obtain sufficiently controllable dyeing rates, they must be applied in a nearly neutral dyebadi. It was found that the application of the 1:2 metal complex dyes was simplified by the addition of weakly polar groups, such as sulfonamide and methylsulfonyl groups, in place of the usual sulfonic acid solubilizing groups.
The ranges of dyes of this type were carefully built up from selected dyes with compatible dyeing behavior and were probably the first range of dyes designed in this way. The previous approach had been to establish large ranges of dyes that were applied by the same general method, and from these ranges compatible groups could be selected by the users.
Fiber-Reactive Dyes.
The reactive dyes, which began to be used commerically in 1956, are increasing in importance so rapidly that they may well become the most important class of dyes in the foreseeable future. All of the dyes that have been discussed so far are reversibly absorbed by the substrate in dyeing. The only exception is the mordant dyes, but even these can be recovered in an unchanged form by appropriate treatment, despite the possibility of chemical linkage between the metal atoms and the substrate. This is not true of the fiber-reactive dyes, which have a dye molecule bearing a labile atom or group; these dyes can form stable covalently bonded compounds between the colored residue of the dye and the fiber.
Fiber-reactive dyes were first investigated in 1894 by the British scientists C. F. Cross and E. J. Bevan, the discoverers of cellulose xanthate, which is an essential intermediate in the manufacture of viscose rayon. They observed that cellulose can be reacted with strong caustic soda to form a cellulose salt called soda cellulose and that this could be readily reacted with various acylating reagents.
Proceeding from this starting point, Cross and Bevan synthesized an azo dye that was chemically linked to cellulose and was faster than the common azo dyes available at that time. However, their process was very complex and of little value industrially. The investigations of the British chemists I. D. Rattee and W. E. Stephen in 1953 produced a highly efficient reaction under mild conditions on the basis of a proper understanding of the physical chemistry of the system. These investigations have been intensively pursued in many countries with the result that fiber-reactive dyes constitute the most important class of dyes for cellulosic fibers.
The main target of current fiber-reactive dye research has been dyes for cellulosic fibers. This is the most important textile fiber, and prior to the development of fiber-reactive dyes it was the most difficult fiber to dye with a compatible dye range covering all shades. Direct cotton dyes based on the azo system were deficient in bright shades and good blues, while vat dyes based on quinone systems provided very poor reds.
There are two reasons for the very rapid expansion in the use of fiber-reactive dyes for cellulose. First, they are very easy to apply and are particularly suitable for application by low-cost, high-production methods. Second, their fastness to wet treatment depends only on the stability of the chemical linkage between the dye and the fiber. Thus, the chromogens—the part of the molecule that determines the dye color—may be selected according to their shade and their stability against direct chemical attack by light, bleach, and other substances. The chromogen may be of any suitable type, such as azo or anthraquinonoid; it may chelate metal atoms; it may even be cationic or non-ionic. Non-ionic reactive dyes have been developed for application to nylon by reaction with the amino groups it contains.
Research has been done on the development of fiber-reactive dyes for wool and nylon with some success. The achievement of high wet fastness on wool and nylon with a wide range of shades is possible using conventional dyes; consequently the technical incentive has been less than in the case of dyes for cellulose. Recent developments in minimum-care wool fabrics are creating the need for very fast wool dyes, and fiber-reactive dyes may well provide the solution to the problem. Current research on the production of fast dyes involves dyes that polymerize on the fiber but do not necessarily react with it.